Ammonia has been used as fertilizer for over a century and has established mature infrastructure for the production capacity, transportation, storage, and distribution network. The global production of ammonia is around 185 million metric tons, and the production capacity is over 195 million metric tons. As a liquid-phase hydrogen carrier, ammonia has drawn attention in recent years due to its high volumetric energy density and low cost. The conventional Haber-Bosch (HB) process consumes natural gas to obtain hydrogen and obtains nitrogen from the air. Ammonia carries more hydrogen than liquid hydrogen in the same volume, ammonia contains 108 gH2/L, and liquid hydrogen has 71 gH2/L. Comparatively, compressed hydrogen only contains 14 gH2/L at 200 Bar. Although the reforming of ammonia back to hydrogen incurs losses, ammonia has the advantage of low transportation costs. The estimated long-distance shipping cost for ammonia is in the range of 1.5~3 US$ per GJ (or equivalent to 0.028~0.056 US$/kg ammonia), compared to typical LNG shipping cost of 1 US$ per GJ. After reforming, the ammonia-derived hydrogen landed cost via shipping is 3~8 US$/kg, depending on source locations.
The international shipping of ammonia is through cryogenic tanks with volumes of from 80,000 to 160,000 m3. The loading and offloading rates vary between 12,000 and 14,000 m3 per hour depending on the tank size. The boil-off rate of ammonia shipping per day is 0.024%, compared with LNG of 0.12% and methanol of 0.005%. During the shipping, ammonia is maintained at -34oC and atmospheric pressure in the tank. The average density of ammonia under such conditions is 682.8 kg/m3.
The conventional Haber-Bosch is an emission-intensive process that emits more than 2 tons of CO2 for 1 ton of ammonia produced. By replacing the hydrogen generation step from SMR to electrolysis, roughly 75% of the CO2 emission can be reduced, and further optimization can be achieved.The minimum theoretical energy required for renewable ammonia is estimated to be 21.3 GJ/t.However, in practise, roughly 38 GJ/t electrical input is consumed in the pilot processes. In a feasibility study conducted by Osman et al. the large-scale continuous operating plant has a specific energy consumption of 10.43 kWh/kg NH3 (equivalent to 37.5 GJ/t, electricity to ammonia efficiency of 49.5%, based on LHV), and the roundtrip electrical efficiency (electricity-ammonia-electricity) is 37.4%.
The cracking process favors high temperature and low pressure. To achieve full decomposition, 400oC is necessary at 1 Bar pressure. With the increase of operating pressure, higher temperature is needed for the full cracking.
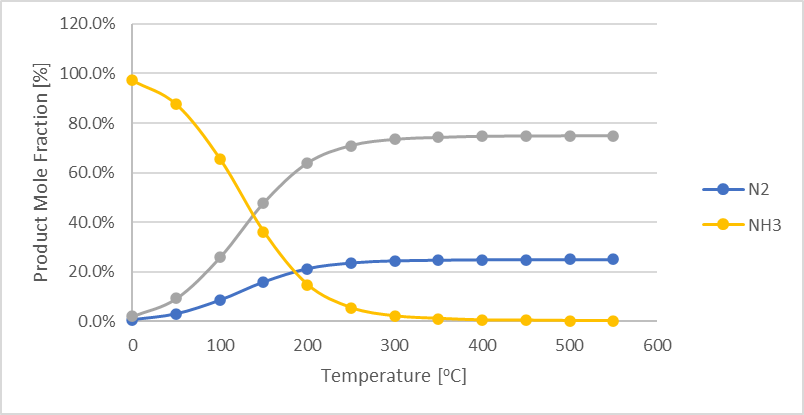
Figure 1 Ammonia cracking thermodynamics at 1 bar and increasing temperature.
The on-site plant consists of ammonia storage system, cracking unit, gas separation, compression, and hydrogen buffer storage. The material flow and main components are illustrated as below.

Figure 2 On-site ammonia cracking process configuration.
The levelised cost of the produced hydrogen is shown below. The main portion of the cost is attributed by the fuel portion (i.e., the ammonia cost). The CAPEX can be levelied down at higher utilization rate of the plant.

Figure 3 Levelized cost of hydrogen with respect to increasing capacity factor
The assumptions for the cost estimation is tabulated as below:

We developed a calculation toolkit for the customizable setup cost estimation. The key parameters and control variables can be changed with user inputs. More options or processes can be added to the interface according to the user's request.
Comentarios